Radioactive Decays Ignite Half of Earth's Heat
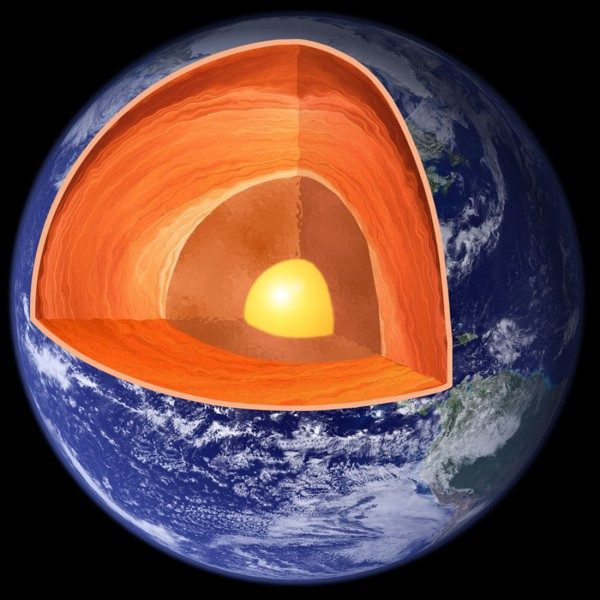
We are aware that heat is the primary driver of our Earth. From the planet's core to its surface, heat enables Earth's magnetic field, spreads the sea floor, and keeps continents on the move.
About 44 terawatts (44 trillion watts) of heat continually flow from Earth's interior into space. But, where does that heat come from?
According to recent research, radioactive decay supplies only about half the Earth's heat, while primordial heat left over from the planet's formation, and possibly others as well - account for the rest.
Radioactive decay of uranium, thorium, and potassium in Earth's crust and mantle is considered as one of the principal source of heat, and in 2005 scientists in the KamLAND collaboration, based in Japan, first showed that there was a way to measure the contribution directly.
KamLAND stands for Kamioka Liquid-scintillator Antineutrino Detector.
The trick was to catch what KamLAND dubbed geoneutrinos - more precisely, geo-antineutrinos - emitted when radioactive isotopes decay.
As a detector of geoneutrinos, KamLAND has distinct advantages, says Stuart Freedman of the U.S. Department of Energy's Lawrence Berkeley National Laboratory (Berkeley Lab), which is a major contributor to KamLAND.
Based on the improved sensitivity of the KamLAND detector, plus several years' worth of additional data, the new estimate is not merely consistent with the predictions of accepted geophysical models but is precise enough to aid in refining those models.
All models of the inner Earth depend on indirect evidence. Leading models of the kind known as bulk silicate Earth (BSE) assume that the mantle and crust contain only lithophiles (rock-loving elements) and the core contains only siderophiles (elements that like to be with iron).
Thus all the heat from radioactive decay comes from the crust and mantle - about eight terawatts from uranium 238 (238U), another eight terawatts from thorium 232 (232Th), and four terawatts from potassium 40 (40K).
KamLAND's detection method is insensitive to the low-energy part of the geoneutrino signal from 238U and 232Th and completely insensitive to 40K antineutrinos, which are the antiparticles of neutrinos.
Neutrinos are neutral particles produced in nuclear beta decay. These are emitted in beta particle emissions, where a neutron turns into a proton.
Other kinds of radioactive decay are also missed by the detector, but compared to uranium, thorium, and potassium are negligible contributors to Earth's heat.
KamLAND detected 841 candidate antineutrino events between March of 2002 and November of 2009, of which about 730 were reactor events or other background. The rest, about 111, were from radioactive decays of uranium and thorium in the Earth.
These results were combined with data from the Borexino experiment at Gran Sasso in Italy to calculate the contribution of uranium and thorium to Earth's heat production. The answer was about 20 terawatts; based on models, another three terawatts were estimated to come from other isotope decays.
This is more heat energy than the most popular BSE model suggests, but still far less than Earth's total.
One thing we can say with near certainty is that radioactive decay alone is not enough to account for Earth's heat energy. Whether the rest is primordial heat or comes from some other source is an unanswered question, says Freedman.
Better models are likely to result when many more geoneutrino detectors are located in different places around the globe, including midocean islands where the crust is thin and local concentrations of radioactivity (not to mention nuclear reactors) are at a minimum.
This is what's called an inverse problem, where you have a lot of information but also a lot of complicated inputs and variables. Sorting those out to arrive at the best explanation among many requires multiple sources of data, Freedman said.
© Copyright IBTimes 2024. All rights reserved.