Nuclear Fusion In Our Time? Scientists Outline Hurdles To Potentially Transformative Energy
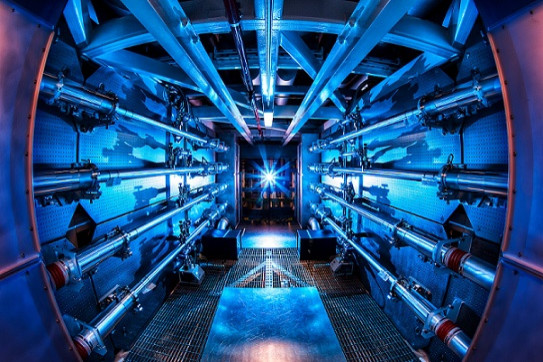
Nuclear fusion, the engine that drives the sun, could potentially transform human society if we could harness it. But researchers at a U.S. government laboratory working toward producing fusion energy are finding that a tiny little component of their apparatus is throwing a very big wrench in the works.
The concept is relatively simple on its face: the nuclei of atoms fuse together and, in the process, release energy -- a lot of energy. A single gram of deuterium and tritium, which are variations of hydrogen, can produce nearly 10 million times the amount of energy from the same amount of fossil fuels, with no side products of greenhouse gases. The radioactive waste products are much less long-lived than the ones produced by current nuclear reactors, and unlike nuclear reactors, there’s no meltdown risk -- if a fusion reactor loses energy, the reactions simply stop. Deuterium can be found in ordinary water, so if fusion’s potential is realized, one gallon of water could provide the energy equivalent of 300 gallons of gasoline, according to the U.S. Department of Energy.
For decades, scientists have struggled to reach “ignition,” the point at which the fusion reaction starts producing more energy than the power put into it. Turns out it’s difficult to heat something to 100 million degrees! In stars, fusion occurs thanks to the intense pressures of gravity; here on Earth, scientists have to find a different approach. In a recent paper appearing in the journal Physics of Plasmas, the staff from the National Ignition Facility at the U.S. government’s Lawrence Livermore National Laboratory reports on their progress toward the ignition point using high-powered laser beams.
The NIF uses a system of 192 laser beams to heat deuterium and tritium atoms held inside a capsule the size of a ball-bearing, which is placed inside a cryogenically cooled, pencil-eraser-sized cylinder called a “hohlraum” (German for “hollow room”). The energy from the laser's pulse subjects the deuterium-tritium fuel to pressures and temperatures approaching the conditions at the center of the sun.
"What we want to do is use the X-rays to blast away the outer layer of the capsule in a very controlled manner, so that the D-T pellet is compressed to just the right conditions to initiate the fusion reaction," NIF associate director John Edwards said in a statement.
The NIF has already gotten pretty close to achieving ignition. The energy is beamed to the right spot, X-rays inside the hohlraum are intense enough, and the compression levels are just about right.
"The demanding requirements set by the ignition point design for targets, diagnostics, and the laser were largely met" in recent experiments, Edwards and his colleagues wrote.
Unfortunately, the capsule that contains the deuterium and tritium keeps breaking apart prematurely.
"In some ignition tests, we measured the scattering of neutrons released and found different strength signals at different spots around the D-T capsule," Edwards says. "This indicates that the shell's surface is not uniformly smooth and that in some places, it's thinner and weaker than in others. In other tests, the spectrum of X-rays emitted indicated that the D-T fuel and capsule were mixing too much -- the results of hydrodynamic instability -- and that can quench the ignition process."
If the team can determine just what’s making the capsule unstable, they can make a sturdier one -- and hopefully progress toward the ignition point.
SOURCE: Edwards et al. “Progress towards ignition on the National Ignition Facility.” Physics of Plasmas 20, 070501 (2013).
© Copyright IBTimes 2024. All rights reserved.