Neutrino Detection: New Simpler, Cheaper Method Proposed
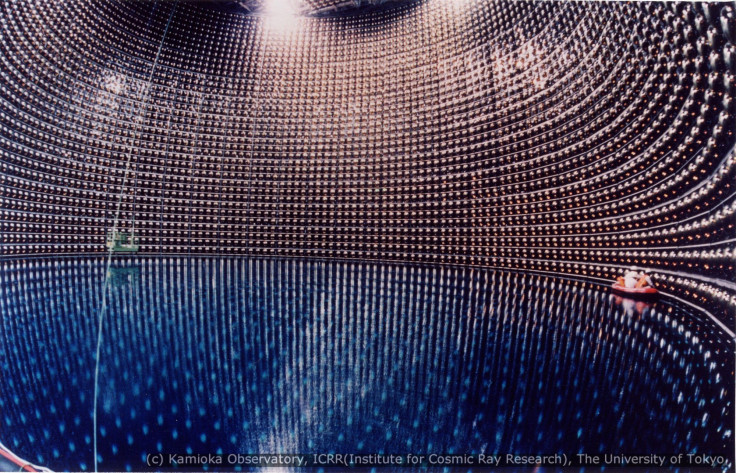
Even though you can’t see, hear, smell, feel or taste them, billions of particles originating from the sun are travelling through your body every second of your life. These tiny particles, called neutrinos, originate due to the nuclear reactions in the sun’s core and due to their unique property of very rarely interacting with any matter, are extremely difficult to detect.
The best existing facility for detecting them is the Super Kamiokande in Japan. Located 1,000 meters (about 0.62 miles) below ground, the observatory has a tank filled with 50,000 tons of ultra-pure water (water with all impurities, both solid and gas, removed from it) which is surrounded by about 13,000 photo-multiplier tubes (extremely sensitive light detectors that work across ultraviolet, visible and near-infrared ranges). If a neutrino passing through the water interacts with electrons or nuclei in it, it causes a charged particle in the water, which moves faster than the speed of light through the liquid. This, in turn, creates an optic shock wave called Cherenkov radiation, which resembles something like a cone of light. This light is recorded by the photo-multiplier tubes.
As is clear from the above explanation, the process is quite complicated and building and maintaining such an observatory is far from cheap (it costs $100 million to build). And studying neutrinos, given their highly elusive nature (even detectors such as the Super Kamiokande can primarily detect only electron neutrinos, one of the three neutrino forms, the other two being muon and tau neutrinos). Which is why the new method proposed by a Stanford solar physicist could really help things along.
Peter Sturrock was inspired by an article on radioactive decay that concerned fluctuations in the rate of decay of radioactive materials, usually considered a constant. Using techniques he developed with Jeff Scargle, astrophysicist and data scientist at NASA Ames Research Center, he decided to study the experimental data of the decay.
Sturrock’s team found a correlation between the fluctuation patterns and the data from the Super Kamiokande, which led them to the likely conclusion that neutrinos from the sun directly affected beta-decays.
In a statement, Sturrock said: “It means there’s another way to study neutrinos that is much simpler and much less expensive than current methods. Some data, some information, you won’t get from beta-decays, but only from experiments like Super-Kamiokande. However, the study of beta-decay variability indicates there is another way to detect neutrinos, one that gives you a different view of neutrinos and of the sun.”
The study of neutrinos can tell us a lot about the interior of the sun, a subject we currently know very little about. That will help our understanding of the early stages of the solar system’s formation. The hunt for neutrinos is also potentially interesting in the search for that other elusive hypothetical particle — dark matter. Scientists theorize a fourth flavor of neutrinos, the sterile neutrino, which could be a candidate for the matter thought to make up the bulk of the observable universe. However, all searches for the sterile neutrino so far have drawn a blank.
The study detailing the findings was published in the journal Solar Physics.
© Copyright IBTimes 2024. All rights reserved.