Can 'Superballistic' Electron Flow Lead To Creation Of Perfect Conductors?
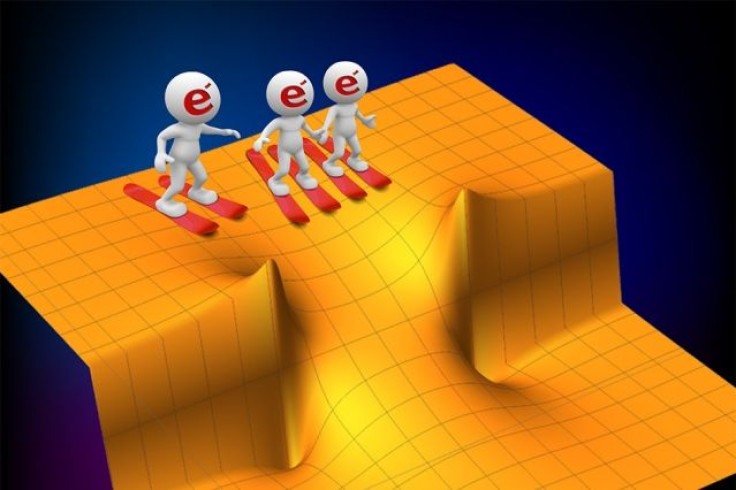
Imagine if we manage to create a material through which electrons move unimpeded, with practically zero resistance. Such a conductor would have an unimaginable range of practical uses — from building electrical grids with lossless power lines to creating highly efficient superconductors and magnetically levitated trains.
But such a material does not exist and the closest real-life analogue of a perfect conductor is a superconductor. Practical applications of these materials are limited by the fact that they need to be cooled to roughly -450 degrees Fahrenheit to function.
In a recent study published in the Proceedings of the National Academy of Sciences, a team of researchers has shown that it is possible — under certain conditions — for electrons to flow through a narrow opening in a piece of metal much faster than previously thought. Moreover, the authors of the study say that this “superballistic” flow of electrons is actually assisted by rising temperature rather than being hindered by it.
“In these constricted passageways, whether for gases passing through a tube or electrons moving through a section of metal that narrows to a point, it turns out that the more, the merrier: Big bunches of gas molecules, or big bunches of electrons, move faster than smaller numbers passing through the same bottleneck,” Massachusetts Institute of Technology (MIT), whose researchers were involved in the study, said in a statement.
The concept is quite counterintuitive. It’s as if a hundred people trying to squeeze through a doorway manage to do so much faster than one person doing it alone.
There is, however, a logical explanation for why this behavior — which has previously been observed in gases flowing through a small opening — occurs. When a lone molecule (or an electron) passes through a constricted passageway, its motion is random, and it loses energy every time it hits the wall. With a bigger batch of particles, most of them will bump into each other more often than they hit the walls, and these collisions are “lossless” — the total energy of the colliding particles is preserved.
“Molecules in a gas can achieve through ‘cooperation’ what they cannot accomplish individually,” lead author Leonid Levitov, a professor of physics at MIT, said in the statement.
For now, the researchers’ model is purely theoretical, and would need to be experimentally tested to see if this superballistic electron flow can be used to drastically improve electrical conductance.
“Testing Levitov's simple and striking predictions experimentally will be really exciting and plausible to achieve in graphene,” David Goldhaber-Gordon, a professor of physics at Stanford University who was not involved in this research, said in the statement. “Researchers have imagined building new types of electronic switches based on ballistic electron flow. Levitov's theoretical insights, if validated experimentally, would be highly relevant to this idea: Superballistic flow could allow these switches to perform better than expected (or could show that they won't work as hoped).”
© Copyright IBTimes 2024. All rights reserved.