Measuring Gravity: MIT Researchers Develop Technique To Increase Accuracy Of Atom Interferometry
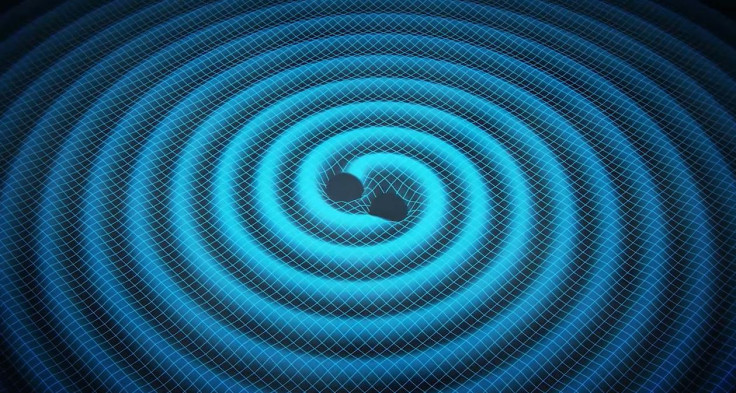
For the longest time, scientists have used a technique known as atom interferometry to carry out extremely precise measurements of gravity — one of the four known fundamental forces of nature. However, although interferometers have enabled us to make very small measurements that wouldn’t have been possible using any other technique, they contain a source of error — one that is the product of an inherent imbalance in an exotic state of matter called the Bose-Einstein condensate.
Named after physicists Satyendra Nath Bose and Albert Einstein, a BEC is a state of matter created when atoms are cooled to a temperature close to absolute zero (0 Kelvin or -459.6 degrees Fahrenheit). At such an ultra-low temperature, all atoms gather in the lowest possible energy state, lose their individual identities, and act as a “giant matter wave” wherein all the atoms inhabit exactly the same quantum state.
It is this unique property that scientists use to create extremely sensitive atom interferometers, which contain a cloud of BEC suspended in a chamber. When a laser beam is fired through this cloud, it creates a “standing wave” — one that divides the condensate into roughly, but not exactly, equal-sized clusters.
“When outside forces act on the condensate, the laser trap keeps them from moving. But when the laser is turned off, the condensates expand, and their quantum state reflects the forces they were subjected to,” researchers from the Massachusetts Institute of Technology (MIT), who have now created a technique to increase the precision of BEC atom interferometers, explained in a statement. “Shining a light through the cloud of atoms produces a visual pattern from which those forces can be calculated.”
The problem with the current technique is that the division of the condensate into separate clusters is often uneven, which can cause errors in measurement. In order to fix this, the researchers used not one, but two different condensates, and, in addition to trapping the condensates with a laser, they also subjected them to a magnetic field.
Doing so ensured that atoms in the condensate naturally shuffled themselves around the standing wave until each “well” between the two zero points of a wave contained exactly the same number of atoms.
“The idea here is that Bose-Einstein condensates are actually pretty big,” William Burton, an MIT graduate student in physics, and lead author of a study detailing the technique, said in a statement. “We know that very small things act quantum, but then big things like you and me don’t act very quantum. So we can see how far apart we can stretch a quantum system and still have it act coherently when we bring it back together. It’s an interesting question.”
© Copyright IBTimes 2024. All rights reserved.