Synthetic Biology Aims To Turn Cells Into Chemists Producing Treatments For Diabetes And Cancer -- And Easing Journeys To Mars
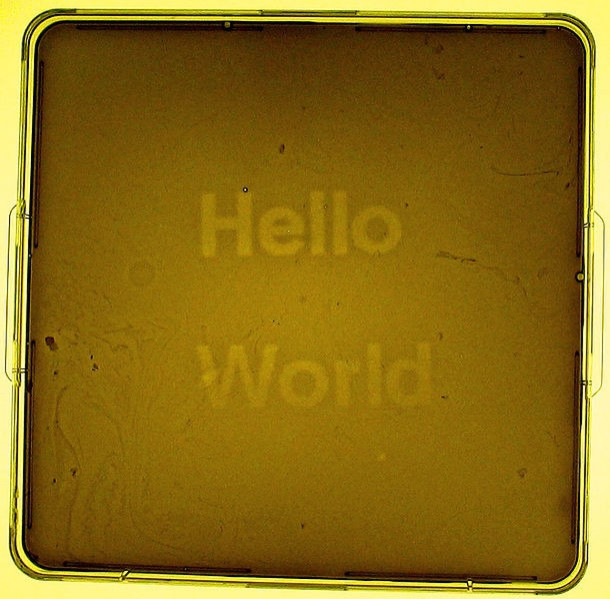
Imagine a diabetic with specially engineered cells that detect the rise and fall of his or her blood sugar and administer insulin if needed. Imagine cancer patients intentionally infected with a "smart virus" that can determine if cells are cancerous and then destroy the dangerous cell from the inside. And imagine cells engineered to excrete biofuels or pharmaceutical products.
These are the visions of synthetic biology. A relatively new breed of researchers, synthetic biologists view the future through a lens in which specially designed DNA sequences, proteins and cells do the work of creating compounds, elements and biological materials that help us treat diseases, produce new kinds of medicines and even establish colonies on other planets.
"The big breakthrough 100 years ago was organic chemistry, when people figured out how to make synthetic materials," says Harvard University biologist Pamela Silver. But "a cell is probably an even better chemist."
The concepts behind synthetic biology have since at the least the 1970s, when scientists inserted the genes to make human insulin into E. coli bacteria, but the outcomes of experiments were notably hit and miss. That's changing quickly.
"Now we're coming to a point where, when I say this part does something, other designers can trust it," said Adam Arkin, a professor of engineering and the director of the University of California at Berkeley's synthetic biology institute.
One project Silver and her colleagues at Harvard are working on is building human and other mammalian cells that can record and remember past events and also be able to "count" forward in time. Such clocked cells would be useful as sensors and could also be part of a new wave of intelligent therapeutics, remembering how a patient's body reacted to previous treatments.
Another project, backed by the U.S. Department of Energy, is a genetically engineered bacteria named Shewanella. Silver and her team are trying to rejigger the bacteria's photosynthetic pathways so it will accept electricity instead of light as an energy source, an advance that would allow biofuel-excreting microorganisms to be plugged into the grid. The Harvard team is also trying to engineer Shewanella to generate fuel that's similar to diesel fuel or gasoline and which requires no additional chemical processing after being pumped out of the bacteria-filled tank.
"It's a very 'blue sky'-type project," Silver says, meaning that Shewanella's success is uncertain.
Synthetic biology's arrival on the scene coincided with the first International Genetically Engineered Machine competition in 2004, in which five teams worked on building their own biological systems. In that initial competition, a team from the University of Texas at Austin created a bacterial photographic "film" using color-changing bacteria that responded to light input. One image captured on this film was two lines of text: "Hello World."
A later image captured with help from the University of California at San Francisco showed the Flying Spaghetti Monster, a mythical beast beloved by many scientists and skeptics. Since then, iGEM teams have created banana- and wintergreen-flavored E. coli, engineered bacteria to find miniscule amounts of arsenic in drinking water and manipulated cellsto intercept the immune system overreaction to infection that causes the often-fatal condition sepsis.
Scientists looking for directions on how to construct their own parts for synthetic biological research can browse an online catalog called the Registry of Standard Biological Parts, which includes specially designed DNA sequences for use in two kinds of bacteria, yeast, a virus called Bacteriophage T7 and mammalian cells.
The field made headlines in May 2010, when the J. Craig Venter Institute announced that it had successfully generated its own version of the genome of the bacterial animal parasite Mycoplama mycoides and inserted that genetic code into a host bacterial cell. Within two days, the M. mycoides cells growing in the lab contained only the synthetic DNA.
At the time, Venter called it "the first self-replicating species we've had on the planet whose parent is a computer," according to the New York Times.
Faster gene sequencing has definitely been a major factor in the acceleration of synthetic biology and will most likely be the key to its commercial viability. The first whole human genome sequence, completed in 2003, took 13 years and $3.8 billion to create. Fast-forward to this past January, when New York-based Life Technologies said it would soon offer genome sequencing in one day, for $1,000.
The exponential cost drop of sequencing and synthesizing DNA means that synthetic biologists can check their work and others' much quicker, all the better to see if a biological part actually does what it is supposed to.
"That changes our cycle times by huge amounts," Arkin says.
On Aug. 15, PLoS ONE published a collection of synthetic biology papers spanning the journal's entire six-year existence. In an introduction to the papers, collection editors Jean Peccoud of Virginia Tech and Mark Isalan of Barcelona's Center for Genomic Regulation said an open-access journal like PLoS ONE was an ideal platform for synthetic biology.
Since the results of synthetic biology research are "often more 'how to build something that works' rather than primary biological insight, the papers can be hard to place in classical journals," Peccoud and Isalan wrote.
Among the papers offered is a November 2009 piece that describes a protein called Gemini, engineered by Stanford and MIT scientists. Gemini was made by combining two kinds of "reporter proteins," which researchers use as a flag to tell them if their gene of interest has successfully integrated into the experimental organism's genome. Another paperfrom Isalan and his colleagues is a catalog of 52 "biobricks" - standardized synthetic biological parts.
Scientists at NASA think there are a host of ways that synthetic biology could play a role in future NASA missions. Plants could be reengineered to tolerate greater stresses and to synthesize their own power receptors, allowing them to grow on harsh Martian (or Jovian, or Plutonian) climates. Astronauts could dine on specially modified bacteria during long spaceflights. Space travelers, specially screened to see if they have the right genetic stuff, could also be dosed with special microbes that would help them cope with long journeys, or perhaps even shield them from radiation.
Plus, a synthetic biology 'toolkit' containing large amounts of genes, enzymes, and proteins wouldn't weigh very much - an important consideration on spaceflights where every extra ounce of baggage adds to the cost of launching.
"For NASA to successfully explore other worlds, we are going to have to take advantage of revolutionary new approaches that synthetic biology affords," researchers from the space agency's synthetic biology initiative wrote in a 2010 workshop paper.
NASA researcher Lynn Rothschild speculated in a paper penned for an astrobiology conference in 2010 that synthetic biology could not only help people cope with alien worlds and space travel, but could also be better equipped to withstand a changing climate on Earth.
"As the Earth itself changes beyond the extremophiles that evolution has produced, synthetic life will be able to leapfrog evolution when the latter moves too slowly to keep up with a changing environment," Rothschild wrote.
But there are still very large hurdles that scientists have to clear for the dream of synthetic biology to become reality.
Synthetic biology tools, it turns out, rarely come in one-size-fits-all packages. As writer Roberta Kwok reported in a 2010 article in the journal Nature, molecular tools are a lot more complicated than hammers and nails and have their own unique challenges. It's hard to standardize biological parts - a gene inserted into a mammal cell can be influenced by the surrounding DNA, and scientists can't control where the gene of interest lands within the genome. And as biological circuits get larger, it becomes harder and harder to make and test them.
"There's a lot of biology that gets in the way of the engineering," Harvard researcher Christina Agapakis told Kwok.
The current catalog of parts is also limited, with the vast majority of components being made for E. coli and baker's yeast. E. coli has performed admirably, but there are some parts it just hasn't evolved to handle, according to Arkin -- it's not like you would put a jet engine in a tiny sedan. More work will be necessary to build parts for more complex hosts, including mammals and plants.
Another problem is less technical and more social. Public perceptions of genetic modification aren't exactly welcoming, as seen by the pushback against GM crops in Europe and the U.S. Food and Drug Administration's reluctance to approve the AquAdvantage salmon, which has genes added from other fish that allow it to grow year-round instead of seasonally.
Silver points out that the public has already accepted invisible forms of bioengineering -- every time you get a vaccine, you're engineering your immune system, she says. She thinks that as synthetic biology advances, having a clear beneficial product will smooth the way toward acceptance.
"With using things as therapies for humans, maybe people will accept those more readily than with GM crops," she says. Timing can play a role too: "If the world is suffering a food crisis, are we then going to be more accepting?"
© Copyright IBTimes 2024. All rights reserved.