Electrons’ Quantum Nature Observed At Temperatures Close To Absolute Zero
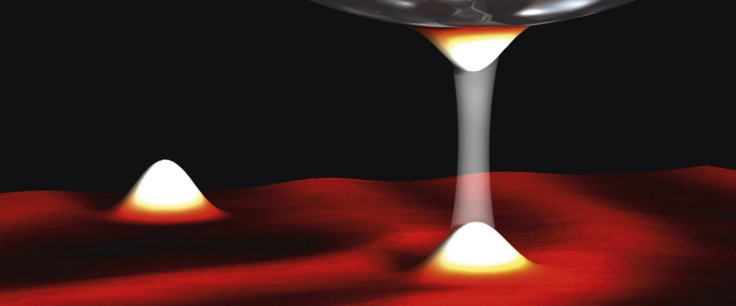
Studying the nature of electrons — the fundamental particles that orbit an atomic nucleus — is something that’s easier said than done. This is because when an electric current flows under room temperature, it is impossible to distinguish individual electrons — even though the laws of physics tell us they are there — much less observe them one at a time.
One way to study the electron’s quantum nature is to slow things down to such an extent that electric current transitions from a homogenous flow to a granular one, where individual electrons trickle through like grains of sand in an hourglass.
“Flowing water from a tap feels like a homogeneous medium — it is impossible to distinguish between the individual water molecules. Exactly the same thing is true about electric current. So many electrons flow in a conventional cable that the current appears to be homogeneous,” researchers from the Max Planck Institute in Germany, who succeeded in observing the full quantum nature of electrons, said in a statement Monday. “Although it is not possible to distinguish individual electrons, quantum mechanics says they should exist. So how do they behave? Under which conditions does the current not flow like water through a tap, but rather trickles like sand in an hourglass?”
In order to do this, the researchers cooled a scanning tunneling microscope — in which a thin, pointed tip scans across the surface of a sample without actually touching it — down to a 15,000th of a degree above absolute zero (–459.65 degrees Fahrenheit). When they did this, they finally discovered an electric current consisting of individual electrons, rather than billions upon billions of electrons that flow every second in a normal current.
At these extremely low temperatures, very fine structures, which the researchers had not expected, became visible.
“We could explain these new structures only by assuming that the tunnelling current is a granular medium and no longer homogeneous,” lead researcher Christian Ast said in the statement. “These extremely low temperatures open up an unexpected richness of detail which allows us to understand superconductivity and light-matter interactions much better.”
© Copyright IBTimes 2024. All rights reserved.